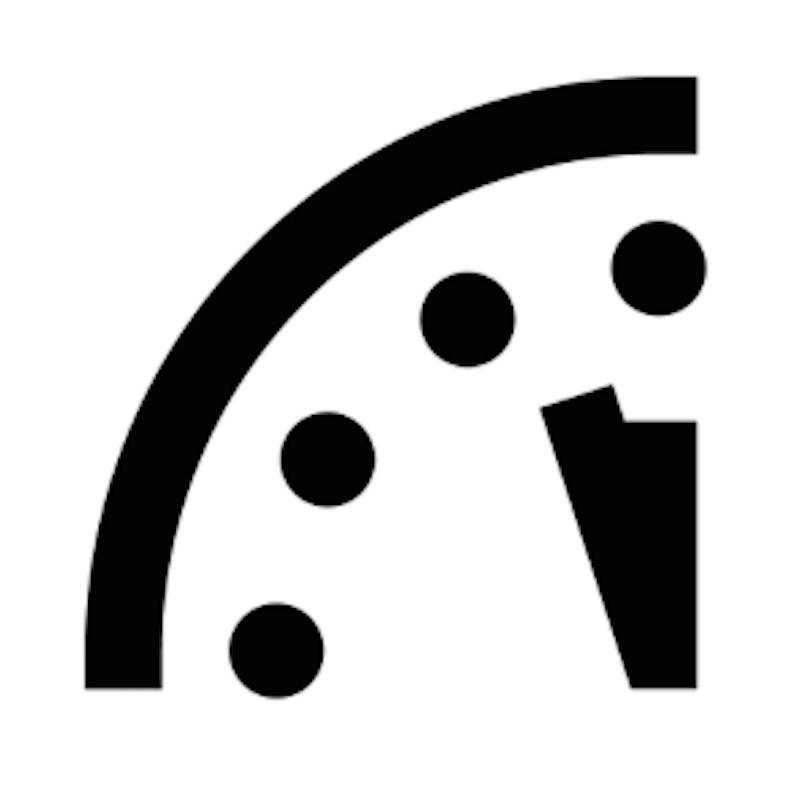
Using nuclear power to replace coal-based fossil fuel power plants worldwide by 2100 is within current international technical capabilities. Whether this can actually be accomplished is however a more complicated matter: The possible obstacles involve negative public perception and fears; dealing with the back end of the nuclear fuel cycle, that is, the ultimate disposal of the used nuclear fuel; and the matter of security, which becomes a potentially far more serious issue as nuclear power plants proliferate. Thus – surely to no thoughtful person's surprise – whether we can realistically replace fossil fuels as a source for electrical generation with nuclear power is a political, and not a technical, issue.
While it is widely recognized that human civilization needs to decarbonize its energy production to counter the ongoing climate impacts of fossil fuel use, there are disparate ideas on how to best achieve this. They range from replacement of all carbon-based energy production technologies to continued reliance on such technologies, coupled with carbon capture and sequestration, or on to some combination of these strategies.
Energy-generation technologies that have a minimal carbon footprint (which we'll call the "green" technologies) include the "renewables" – such as hydro, wind, and solar power – and nuclear power, which currently means energy derived from fission. (We ignore fusion-based nuclear power, as yet unproven as a reliable energy source, in this article.)
What role could nuclear power play in decarbonizing the world's energy (and in particular, electricity) production? This topic has been visited in depth by others through the years, for example by Princeton University nuclear expert Alex Glaser and Princeton senior research scholar Rob Socolow (2009). [1] Here, we examine what is plausible, based on current experience in building nuclear power plants. But, we do not address questions such as the relative merits of hydro, wind, solar, and nuclear power, the various "spent" nuclear fuel issues, and issues of safety and security. Many of these issues were discussed in depth by Glaser and Socolow and have not changed in any substantial way since then.
We also do not address the economic issues involved in a transformation of electricity production that greatly reduces fossil fuel use and increases focus on low- and no-carbon energy sources. We do note that the levelized cost of electricity – that is the cost of electricity taking all investments and operational expenses into account [2] – for new nuclear builds is currently higher than that for either coal or gas in the United States. This cost equation does not hold, however, if the process of carbon capture and sequestration is mandated to allow these fossil-fueled technologies to survive.
At this point, it is useful to ask whether we will contend that nuclear power, on its own, could be the sole solution to "decarbonizing" the electric power sector. Our simple answer is "no," for the following reasons: First, an important issue for grid-scale electricity production is dealing with fluctuations in both supply and demand. Such fluctuations occur on a variety of time scales, ranging from seconds to hours, and different technologies are required to deal with such fluctuations, depending on their time scale. Utilities generally use the most reliable power source for base electricity production[3] and depend on so-called dispatchable electric power sources to deal with the fluctuations.
Nuclear power is widely understood to be well suited for providing base power because its capacity factor – that is, the actual output compared to its potential output if it were to operate at full capacity – is roughly 90 percent in the United States, substantially higher than any competing energy production technology. [4] In contrast, nuclear power is poorly suited for dealing with power fluctuations on the grid (in either supply or demand). For example, on longer time scales (e.g. fluctuations on time scales of tens of minutes to hours, typically driven by variations in demand), utilities currently primarily rely upon gas-fired power plants (often referred to as "peakers") to balance load with supply. In contrast, fluctuations on shorter time scales (down to seconds) can typically result from fluctuations in supply from renewables such as wind and photovoltaics.
The backup power to deal with these rapid fluctuations must have the capability for fast ramp up/ramp down (on time scales as short as seconds), again a characteristic that nuclear power plants do not have. Technologies that do have that capability are predominantly grid-based storage systems such as grid-scale batteries, compressed gas facilities, and a variety of so-called spinning reserves (which can include spinning turbines powered by water or natural gas that are kept off-line until needed) – all instances of reserve power technologies that add substantially to the ultimately delivered cost of electricity. [5]
What all of this implies is that, in a world that has completely "decarbonized" its electricity production, there will be a need for one or more energy generation technologies that can solve the dispatch problem (e.g. supply must always equal load) on a broad range of time scales, and exactly what these technologies will turn out to be remains to be seen. Candidates range from renewables such as wind, hydro, and solar to a variety of storage technologies. But, it will not be nuclear power, and for this reason alone, nuclear power can only be a partial solution to eliminating carbon emissions into the atmosphere. Our future electric grid will require a mix of "green" technologies to fulfill all of the supply requirements typically faced by electric utilities.
Finally, we note that in order to approach zero net carbon input to the Earth's atmosphere as a result of electricity generation, one would need to replace not only the existing complement of fossil-fueled power plants – those plants fueled by coal, petroleum, and natural gas – but also deal with replacement of existing "green" power plants that have reached the end of their service lives. The vast majority of such aged plants are nuclear power plants; wind and solar power have not been around long enough for such retirement to matter, while hydro power plants tend to have very long service lives that can be de facto extended indefinitely via regular surveillance and maintenance. In contrast, there are no presently known methods for preventing physical damage to major nuclear power plant components (such as the reactor pressure vessel due to neutron irradiation) over time.
Because replacement of a reactor pressure vessel is far too expensive to be economically feasible, current expert judgment does not support operating license renewals for nuclear power plants past a nominal 60-year lifespan (American Physical Society 2013). This means that by (at the latest) mid-century, the approximately 100 existing US nuclear power plants would have to be replaced by non-carbon-emitting power generation technology just to maintain the present rate of carbon emission in the United States. Similar reasoning of course applies to the nuclear power plants in other nations, meaning that to maintain the current "green" energy contribution of nuclear power, all of the existing plants would have to be replaced by the end of this century or have their output replaced by other low-carbon sources.
What has to be replaced? What has to be built?
Estimating the amount of fossil fuel-based power that needs to be replaced by green energy production technologies is clearly an exercise in approximation. To start with, we simply aim to get a sense of the magnitude of what would need to be done. We begin with the most recent available numbers in The World Factbook,[6] which provides estimates for the installed generating capacity for all 214 nations/independent governmental units in the world. The worldwide total estimated installed generating capacity in the period 2012–2014 turns out to be approximately 6800 gigawatts. If we adopt the International Energy Agency estimate for the fraction of installed generating capacity attributable to coal (40.4 percent) for this time period, then 2750 GW of installed generating capacity can be attributed to coal. Assuming the 41 percent capacity factor attributed to coal plants by the EIA, this works out to approximately 1230 GW of actual generating capacity that will need to be replaced.
We also need to account for replacing all of the existing nuclear power plants; again erring on the conservative side, we will assume that the operating capacity for the current US nuclear fleet (approximately 90 percent) also applies to all other nuclear plants throughout the world.[7] With this assumption, we obtain a nuclear generating capacity worldwide of 380 GW that will need to be replaced as well. Of course, we will also need to account for growth in energy demand – a subject that is highly controversial, basically because the impacts of increased efficiency in energy use are largely a matter of guesswork.[8] Assuming 50 percent growth in demand over the balance of this century attributable to coal alone (i.e. we assume that the growth in coal-based electricity generation will be substantially lower than that of electricity demand overall), we find that a plausible estimate of the total amount of installed green generating capacity to be built by 2100 is of the order of 2200 GW.
Another – and very different – way of thinking about this problem is to ask how many nuclear plants one would need to build in order to have a substantive impact on the global input of carbon into the atmosphere. Princeton professor of ecology and evolutionary biology Stephen Pacala and Socolow (2004) approached this question more generally, asking what would need to be done to keep the carbon dioxide load of Earth's atmosphere at or below 500 parts per million (ppm), a level many experts believe could avert some of the most catastrophic impacts of climate change.
Pacala and Socolow identified 15 possible stabilization scenarios[9] – each capable of reducing carbon emissions by 2054 by one gigaton of carbon per year – and showed that a mix of roughly seven to eight of these "stabilization wedges" could suffice to achieve the goal of limiting carbon dioxide levels to below 500 ppm. One of these "wedges" involved replacing all coal power plants with nuclear power plants. Estimating exactly how many nuclear power plants would need to be built is an exercise in approximation, and for present purposes, we will adopt the estimate provided a bit later on by Socolow and Glaser (2009), namely that approximately 1500 GW of newly built nuclear power plants would constitute one of the abovementioned wedges, an estimate that is somewhat smaller than our above estimate for replacing all fossil fuel plants by 2100. Note that the world would still need approximately six additional wedges to meet Pacala and Socolow's goal of bounding the atmosphere's carbon load – so the single "nuclear wedge" we are discussing is clearly only a partial solution to the climate change challenge. But, this still leaves us asking: Is this single "nuclear wedge" a realistic target for building new nuclear power plants within this century?
How quickly can the world build?
To estimate what could be done in replacing both fossil fuel power plants and retired nuclear power plants with new nuclear power, we looked to the historical record of reactor construction. For obvious reasons – including a history of long construction delays – predicting future build rates for nuclear power plants is a risky proposition; we will therefore err on the very conservative side and not assume build rates that surpass what was accomplished in the past.
According to the International Atomic Energy Agency (IAEA),[11] as of 2016, there are 449 reactors in operation worldwide. The vast majority of these reactors were built in the period 1960–1980, during which 222 reactors were put into operation; in the most active period, 1970–1979, the IAEA's data show that 139 reactors were put in service. So, we will assume that it should be possible to replicate that figure, that is, we shall conservatively assume that a build rate of 14 reactors per year worldwide can be attained over periods of decades in the present century.
Of course, the capability and capacity of modern reactors far exceed what was built during the most active earlier construction period. Generation III+ reactors such as the Westinghouse/Toshiba AP1000 and the Korea Electric Power Corp. (KEPCO) APR-1400 are capable of supplying 1000 and 1400 MWe, respectively, with substantially greater operational safety margins than the plants built in the 1970s, largely because of the introduction of passive safety features absent in earlier generation reactors.[12] It is also evident (and should be obvious) that construction experience matters in terms of getting nuclear reactors built on cost and schedule: Both the South Koreans – in Abu Dhabi, where the Korean consortium building APR-1400 reactors is bringing in the first of four reactors online in 2017[13] – and the Chinese – in their remarkable wave of new reactor construction – have demonstrated that such reactors can be built on budget and on schedule, as long as one has access to a highly trained workforce. (This is certainly in contrast with the recent experience of reactor construction in Finland[14] and in the United States,[15] where lack of experience has resulted in both schedule and cost overruns.)
At the projected build rate of 14 plants per year, and assuming that future reactors are of the Gen III+ variety, each with a plate electric power rating of 1400 MWe, we can expect about 20 GWe added capacity per year based on nuclear power. This means that one could construct one of Pacala and Socolow's earlier discussed "wedges" (which we assumed constituted about 1500 GWe) in 75 years, or about 10 years short of the end of this century. Reaching our yet more ambitious target of 2200 GW would instead take 110 years, a much more sobering projection as it falls short of our goal by a quarter of a century.
But, we again emphasize that our estimates are very conservative: They assume no further advances in nuclear power plant designs and build rates that do not exceed what has already been demonstrated in the distant past, some 30+ years ago.[16] Of course, to meet Socolow and his collaborators' more challenging goal of attaining the requisite mix of wedges by 2050 (or, alternatively, halving the time scale to reach 2200 GW), and assuming no advances in reactor design, we would need a build rate roughly twice as large as assumed, that is, approximately 28 reactors per year. Such a build rate substantially exceeds what has been achieved to date, and we will only know if this can be achieved by observing the ongoing nuclear construction boom in nations such as China.
Of policy and politics
Our brief discussion should make plain that using nuclear power to replace coal-based fossil fuel power plants worldwide by 2100 – or, roughly equivalently, constructing one of the "wedges" discussed by Socolow and collaborators by 2100 – is within current international technical capabilities. Whether this can actually be accomplished is however a more complicated matter: The possible – and actually very likely – obstacles involve precisely the issues we've avoided discussing: first, the matter of negative public perception and fears; second, dealing with the back end of the nuclear fuel cycle, that is, the ultimate disposal of the used nuclear fuel; and third, the matter of security, which becomes a potentially far more serious issue as nuclear power plants proliferate. Thus – surely to no thoughtful person's surprise – whether the world can realistically replace fossil fuels as a source for electrical generation with nuclear power is ultimately a political, and not a technical, issue.
Disclosure statement
No potential conflict of interest was reported by the authors.
Funding
Support for the editing of this article was provided by the Pulitzer Center on Crisis Reporting.
Notes
1. See also Brock, B.W. (2011), http://dx.doi.org/10.1016/j.enpol.2011.11.041, and Qvist S.A., Brook B.W. (2015) Potential for Worldwide Displacement of Fossil-Fuel Electricity by Nuclear Energy in Three Decades Based on Extrapolation of Regional Deployment Data. PLoS ONE 10(5): e0124074. doi:10.1371/journal. pone.0124074. These authors offer a much more optimistic view; we have instead opted for a decidedly conservative approach. See also the Secretary of Energy Advisory Board (SEAB) Report of the Task Force on the Future of Nuclear Power (September 22, 2016).
2. See https://www.eia.gov/forecasts/aeo/pdf/electricity_generation.pdf.
3. The "base load" refers to the minimum demand level on an electrical grid over a daily cycle.
4. For example, fossil fuel power has a capacity factor of about 41 percent, hydro-based power has a capacity factor of approximately 40 percent, and wind and solar follow with approximately 27 percent; these percentages reflect the average capacity factor during the 2008–2012 period; see http://www.eia.gov/todayinenergy/detail.php?id=22832.
5. This is one of the reasons that the usually quoted cost of electricity provided by these renewable technologies is an unreliable indicator of the actual cost of electricity produced by these "green" technologies; they ignore the need for this type of backup power.
6. See https://www.cia.gov/library/publications/the-world-factbook/rankorder/2236rank.html.
7. That is, by doing so, we overestimate the needed number of new nuclear power plants to be built since the operating capacity of existing nuclear power plants outside the United States tends to be well below 90 percent.
8. For example, contrast the projection http://www.eia.gov/todayinenergy/detail.php?id=12251s by the BP Energy Outlook (www.bp.com/energyoutlook) with projections by the U.S. EIA for 2040 (http://www.eia.gov/todayinenergy/detail.php?id=12251).
9. Pacala and Socolow refer to these scenarios as "wedges," in the spirit of wedges or slices of a pie chart, the idea being that 7–8 of these "wedges" would add up to a complete pie that would fully cap carbon emissions by 2054. Examples of such "wedges" include increased vehicle efficiency (viz. increasing fuel economy for two billion cars from 30 to 60 mpg) and replacing all coal power plants with wind power (viz., one million one MW-peak wind mills).
10. Note that Socolow and Glaser (2009, Socolow, R. H., and A. Glaser. 2009. "Balancing Risks: Nuclear Energy & Climate Change." Daedalus 138: 31–44. [CrossRef], [Web of Science ®]) somewhat revised the definition of a "wedge," requiring it to avoid four billion tons/year of carbon dioxide emission by 2050, versus one billion tons/year of carbon emission in 2054.
11. See https://www.iaea.org/PRIS/WorldStatistics/OperationalReactorsByCountry.aspx.
12. See, for example, Nuclear Reactors: Generation to Generation, Goldberg, S.M., & Rosner, R. 2011, Occasional Papers Series, American Academy of Arts & Sciences, pp. 1–25.
13. See Nuclear Power in the United Arab Emirates (2016), in Information Papers: Country Briefings (World Nuclear Association [WNA]), July 2016.
14. See, for example, World Nuclear News, issue of 1 September 2014.
15. See http://www.nytimes.com/2016/02/09/us/politics/half-built-nuclear-fuel-plant-in-south-carolina-faces-test-on-its-future.html.
16. The one technical issue is that the current worldwide capacity for providing the very large forgings needed for constructing the pressure containment vessel of gigawatt-class nuclear reactors would be challenged by such build rates. However, this is not a technological roadblock, but rather a matter of economics, since it is likely that the commercial forging capacity would respond to demand.
Additional author information
Robert Rosner
Robert Rosner is the William E. Wrather Distinguished Service Professor in the Departments of Astronomy & Astrophysics and Physics at the University of Chicago. Rosner recently stepped down as Director of Argonne National Laboratory, where he had also served as Chief Scientist. His research is mostly in the areas of computational plasma physics and fluid dynamics (focusing on both laboratory and astrophysics applications) and more recently has branched out into aspects of energy policy.
Alex Hearn
Alex Hearn is an undergraduate at the University of Chicago, pursuing a bachelor's degree in public policy with a specialization in nuclear energy. His research interests include decommissioning nuclear power plants, high-level-nuclear-waste management, the psychical impact of radiation, and international cooperation.